Plate Tectonics Overview
Translations: Romanian translation , Ukrainian, Swedish, Russian, Indonesian, Irish
CONTINENTS ADRIFT
During the 19th and early 20th century, several geologists explored the idea that the continents may have moved across the Earth's surface. They were all inspired by the remarkable fit between the Atlantic coasts of Africa and South America. The hypothesis of continental drift was largely developed by the German Alfred L. Wegener, a lecturer in astronomy and meteorology, who suggested that the Earth's continents had at one time been joined in two supercontinents. In the year 1912, Wegener made the proposal that all the continents were previously one large continent, but then broke apart, and had drifted through the ocean floor to where they are now located. Apart from using the fit of the two continents already mentioned, Wegener also used fossil distribution and lithological similarity as evidence. Wegener was born in Berlin on November 1, 1880 the youngest child of an evangelical preacher. By the time he was in his teens, he had developed a strong interest in the earth sciences. He studied astronomy at the University of Berlin, where he received a doctoral degree in 1904. Wegener exhibited a talent for expounding complex subjects with great ease. Together with the force of his personality, the clarity of Wegener's vision inspired great enthusiasm and loyalty among his students.
Of course, the "Drift" theory was not immediately accepted by Wegener's peers, as it is difficult in the world of science to change accepted or established doctrines or views. Two other viewpoints prevailed at this time. Those who believed that the continents and basins were basically unchanged in their position and relative configuration since they were formed were called "Permanentists". Others believed that as a result of the gradual contraction of the solid earth, ocean floor became dry land, and dry land in turn became ocean floor; these scientists were called "Contractionists".
Wegener studied the distribution of animals and fossil land plants to help him in his interpretations. Wegener found that the plant Glossopteris had left behind leaf remains which were relatively common in the Southern Hemisphere continents. This supported his hypothesis, as Wegener reasoned that in order for Glossopteris leaves to be found in the widely spaced continents of the Southern Hemisphere, the continents must once have been joined. Using this evidence, he joined all of the southern continents, together with India, into a supercontinent which he named Pangea.
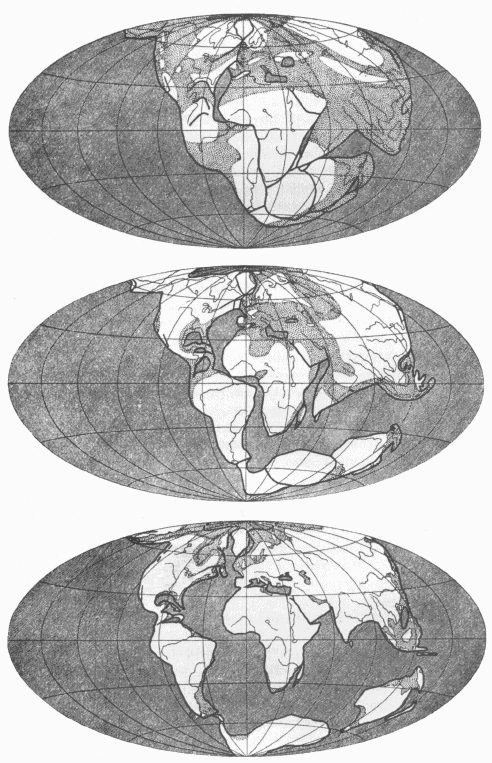
Lithology
Wegener also studied the distribution of major geological bodies, such as crystalline basement (rocks and continental crust) complexes and mineral deposits. He found that the fit predicted by map estimates was confirmed by the alignment of geological complexes on either side of the Atlantic Ocean. For instance when he fitted Africa and South America together along their continental shelves, he found that large blocks of ancient rock called cratons formed contiguous patterns across the dividing line. The mountains that run from east to west across South Africa seemed to link with the range near Buenos Aires in Argentina. The distinctive rock strata of the Karoo system in South Africa, which consists of layers of sandstone, shale and clay laced with seams of coal, were identical to those of the Santa Catarina system in Brazil.
-
Fossil Distribution
South Africa's contribution
Wegener's most compelling and enthusiastic support came from a South African geologist, Alexander Du Toit. South African scientists were far more favorably disposed to the idea of continenal drift for a simple reason: All around them they could see a plethora of geological phenomena that closely resembled those of the other continents in the Southern Hemisphere. Du Toit spent five months in Brazil, Uruguay and Argentina amassing evidence. He found it difficult to believe that he was on another continent as not only did he find the same plant and animal fossils he knew at home, but he found them in the same complex sequence, embedded layer by layer in the rock. Du Toit was confident he had found conclusive proof that the continents were once joined. In a book dedicated to Wegener and entitled Our Wandering Continents, Du Toit proposed a prior configuration for the continents that was different from Wegener's.
Instead of a simple supercontinent, Du Toit reconstructed the continents at the South Pole and grouped the northern continents near the Equator. He called his southern supercontinent Gondwanaland and the northern land mass Laurasia. He devoted most of his book to Gondwanaland and as evidence for its existence he produced an impressive mass of data far more detailed than anything Wegener had attempted.
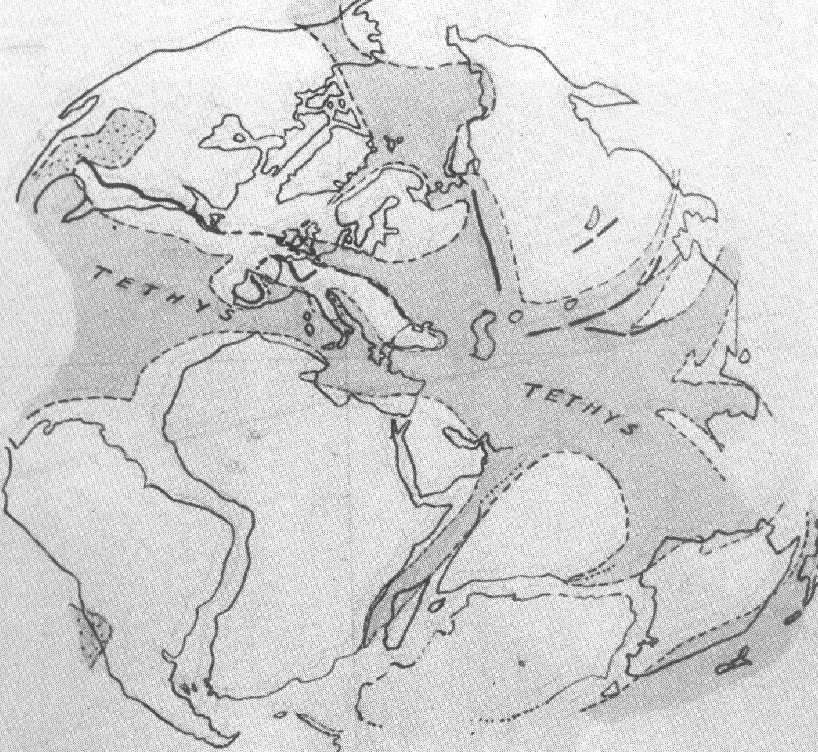
Was this good enough for the academic critics ? No. Du Toit's flamboyant writing style pained the critics. For instance he would write "the dumbfounding spectacle of the present continental masses, firmly anchored to a plastic foundation yet remaining fixed in space; set thousands of kilometers apart, it may be, yet behaving in almost identical fashion from epoch to epoch and stage to stage like soldiers at a drill; widely stretched in some quarters at various times and astoundingly compressed in others, yet retaining their general shapes, positions and orientations; remote from one another throughout history, yet showing in their fossil remains common or allied forms of terrestrial life; possessed during certain epochs of climates that may have ranged from glacial to torrid or pluvial to arid, though contrary to meteorological principles when their existing geographic positions are considered - to mention but a few such paradoxes!" Du Toit's overdramatization succeeded only in decreasing the value of his substantial contributions to the evidence. "This," sniffed an academic critic, "is the colorful language of a pamphleteer."
How the critics were converted
The discovery of palaeomagnetism and the development of oceanography was a necessary step in the development of science which Wegener's and Du Toit's theories awaited.
Paleomagnetism
Paleomagnetism is based on the principle that in molten igneous rocks, or unlithified sediments, magnetic particles will align themselves with the Earths's magnetic field. This magnetic record is stored within the rocks when they cool and within the sediments when they become lithified. The deviations in the alignment of these paleomagnetic particles from the current direction of the Earth's magnetic filed shows that the continents have moved. A British physicist Patrick Blackett, who had won the Nobel Prize in 1948 for his work in nuclear physics and cosmic radioation, developed a sensitive device called the astatic magnetometer. Using this equipment, it was possible for the first time to detect the orientation of extremely weak magnetic fields. This enabled researchers to conduct paleomagnetic studies of types of rocks whose magnetism could not be discerned by earlier equipment.
Oceanography
During the 1960's two Cambridge scientists, Drummond Matthews and Fred Vine discovered that on either side of the Mid-Atlantic Ridge there were a series of linear magnetic anomalies. Strips of ocean crust had alternating magnetic orientations. These observations were explained in terms of a sea floor spreading model by which new oceanic crust forms along mid-ocean ridges as the two halves of an ocean move apart.
From these simple observations the theory of PLATE TECTONICS developed.
Plate TectonicsAccording to the plate tectonic model, the surface of the Earth consists of a series of relatively thin, but rigid, plates which are in constant motion. The surface layer of each plate is composed of oceanic crust, continental crust or a combination of both. The lower part consists of the rigid upper layer of the Earth's mantle. The rigid plates pass gradually downwards into the plastic (soft) layer of the mantle, the astenosphere. The plates may be up to 70 km thick if composed of oceanic crust or 150 km incorporating continental crust. Plates move at different velocities, The African plate moves about 25 mm per year, whereas the Australian plate moves about 60 mm per year.
Most of the Earth's tectonic, seismic and volcanic activity occurs at the boundaries of neighbouring plates. There are three type of plate boundaries: divergent, convergent and transform boundaries.
Divergent plate margins
At this type of boundary new oceanic crust is formed in the gap between two diverging plates. Plate area is increased as the plates move apart. Plate movement takes place laterally away from the plate boundary, which is normall marked by a rise or a ridge. The ridge or rise may be offset by a transform fault. Presently, most divergent margins occur along the central zone of the world's major ocean basins. The process by which the plates move apart is referred to as sea floor spreading. The Mid-Atlantic Ridge and East Pacific Rise provide good examples of this type of plate margin.
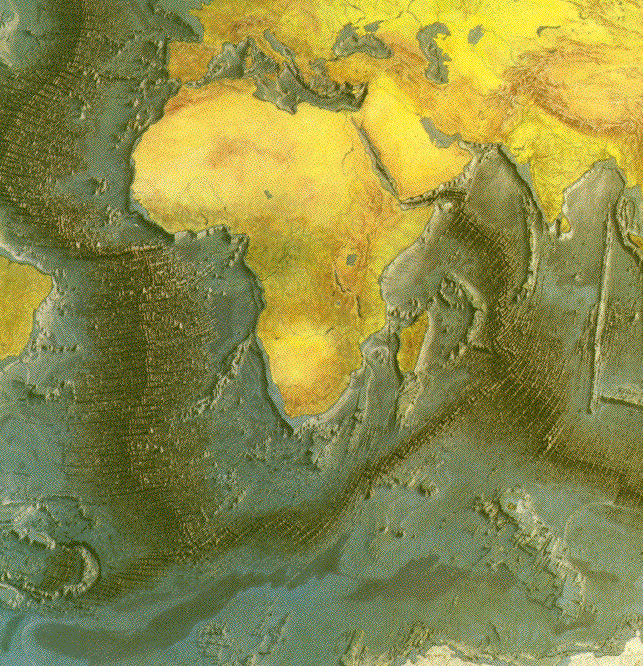
Convergent plate boundaries
At a convergent boundary two plates are in relative motion towards each other. One of the two plates slides down below the other at an angle of around 45 degrees and is incorporated into the Earth's mantle along a subduction zone. The path of this descending plate can be found from analysis of deep earthquakes and the initial point of descent is marked on the surface by a deep ocean trench . Plate area is reduced along the subduction zone. When two plates of oceanic crust collide a volcanic island arc may form. As one of the plates is subducted beneath the other it begins to melt at a depth of between 90 and 150 km and the resulting magma rises to the surface above the subduction zone to form a chain or arc of volcanoes. The edge of the plate which is not descending is therefore marked by a chain of volcanic islands.
Conservative or transform margins
The San Andreas fault system is the most famous example of this type of boundary. Here two plates move laterally past each other and oceanic crust is neither created nor destroyed.
-
The rate at which each plate moves apart from a divergent margin varies from less than 50 mm per year to over 90 mm per year and can be determined from the pattern of magnetic anomalies either side of a spreading ridge. Either side of a spreading centre, weak magnetic anomalies 5-50 km wide and hundreds of kilometres long can be identified. molten rock cools between diverging plates the magnetic minerals present align themselves with the orientation of the Earth's magnetic field at that time. The polarity of the Earth has changed at regular intervals throughout geological time. Magnetic north has alternated between the Arctic (normal polarity) and the Antarctic (reversed polarity). As a result of this, sections of crust formed during a period of normal polarity have a paleomagnetic remnance which is oriented towards today's magnetic north, while a section of crust formed during a period of reversed polarity does not. These long linear strips of magnetic anomalies form a symmerical pattern either side of a spreading centre. A record of the changes in the Earth's magnetic polarity has been established and dated for the Cenozoic and is the basis for magnetostratigraphy. This record, in conjunction with the magnetic stripes found either side of a spreading ridge, allows the rate and pattern of sea floor spreading to be examined.
What causes plates to move ?
This question has yet to be fully resolved. Four main hypotheses have been put forward to explain this.
Convection currents
This hypothesis suggests that flow in the mantle is induced by convection currents which drag and move the lithospheric plates above the astenosphere. Convection currents rise and spread below divergent plate boundaries and converge and descend along convergent. Three sources of heat produce the convection currents:
(1) cooling of the Earth's core
(2) radioactivity within the mantle and crust
(3) cooling of the mantle
The convection hypothesis has been proposed in several different forms throughout the last 60 years. Convective models of plate evolution clearly show how important convective heat transport is to the modern Earth, over length scales as small as 100 km and times of 60 million years. Earth is a spendthrift, living on its inherited capital of primaeval heat, not on its radiogenic modern income.
Magma injection This hypothesis invokes the injection of magma at a spreading centre pushing plates apart and thereby causing plate movement.
Gravity Oceanic lithosphere thickens as it moves away from a spreading centre and cools, a configurationwhich might tend to induce plates to slide under the force of gravity, from a divergent margin towards a convergent margin.
Descending plates This hypothesis suggests that a cold dense plate descending into the mantle at a subduction zone may pull the rest of the plate with it and thus cause plate motion.
To summarize, the plate tectonic model provides a mechanism by which:
(1) continents can move across the surface of the globe
(2) patterns of volcanism can change and shift across the globe as plates and their boundaries evolve and move
(3) new oceans may grow and different sedimentary basins evolve
(4) oceans and sedimentary basins close and are deformed to produce mountains
Do measurements using VLBI, SLR and GPS support the findings from paleomagnetism ?
Yes, it does.
Geodetic data from VLBI, SLR and GPS indicate that plate velocities as measured over the last 15 years nearly equal those averaged over the past 3 million years.
For more information on plate tectonics, read